
Antibiotic Resistance: Fighting a Multi-Headed Demon
Posted On Monday, March 04, 2019 by Abhinav Choudhry under Sustainable Development Uncategorized
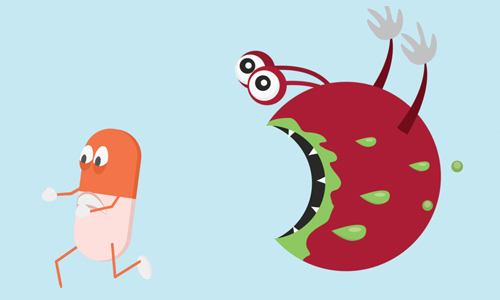
Written by Abhinav Choudhry
The 1928 discovery of penicillin was probably the 20th century’s singular medical discovery with the greatest impact, not only directly saving millions of lives but spawning an entire new field of antibiotics. Naturally occurring substances from soil could suddenly be harnessed to cure bacterial origin diseases almost magically. Yet, the discoverer, Alexander Fleming, presciently cautioned against the mismanagement of this precious resource and noted how inadequate and improper dosage could selectively leave some bacteria to become ‘educated’ against penicillin, eventually causing the diseases to become uncurable (NY Times 1945). This ominous phenomenon of antibiotic resistance has since been noticed for all antibiotics developed since then and caused tremendous heartburn for patients, scientists and public health officials across the world. Many fear a future in which hitherto easily treatable bacterial diseases such as pneumonia and tuberculosis (TB) would once again be unassailable. Thus, there has been considerable furore over relative inaction on preventable causes of antibiotic resistance, especially concerning the use of antibiotics in livestock agriculture.
Origins
The ‘Cassandras’ have strong grounds. Bacteria could both be fearsome adversaries and trusted allies comprising about one twentieth of our body weight. Bacteria living on the skin and insides of mammals have a symbiotic relationship because it facilitates their proliferation (Michael and Plotkin 2002). Mammalian surfaces are far preferable to the vicissitudes of the natural environment. When humans arrogantly use antibiotics to wipe out bacteria , they forget that antibiotics may have evolved as signaling molecules used by bacteria (Salyers 1995). Antibiotic resistance genes are prehistoric, having been found in 30000-year-old permafrost (D’Costa et al. 2011) and in a cave isolated for 4 million years (Bhullar et al. 2012). They are natural survival tools of the world’s oldest living organisms.
Antibiotic resistance can not only occur through genetic mutation in a bacterial population against a particular antibiotic, but it can occur through horizontal transfer of genes across species through the use of a small DNA molecule called a plasmid. Even worse, the exchange of a DNA segment containing a cluster of resistance genes, or the acquisition of an efflux pump (basically a pumping mechanism that can pump out multiple types of antibiotics from within the bacteria) could confer resistance to multiple classes of antibiotics in one stroke (Salyers 1995). Once resistance develops, resistance levels rapidly become prevalent in the population. These mechanisms have led to the emergence of multiple drug-resistant (MDR), extensively drug-resistant (XDR) and pan-drug resistant bacteria (PDR). XDR bacteria are only susceptible to one or two categories of antibiotics while PDR bacteria are susceptible to none (Magiorakos et al. 2012). Fortunately, combinations of antibiotics still work against XDR and PDR bacteria (Falagas et al. 2005).
Dangers
This ‘fortune’ of some treatment still working is far from costless and is already causing the healthcare sector financial hardship. Prevalent resistance renders empiric therapy (symptom-based) ineffective and necessitates additional testing, longer duration of treatment including hospitalizations and the need for more specialists. First-line broad spectrum antibiotics are cheap and have few ill-effects but stronger drugs are toxic as well as extremely expensive. Resistance also leads to greater drug doses even in successful cases and related toxicity; the side effects of combinations of toxic drugs may not always be easily predictable. The cost and toxicity of last of the line drugs such as colistin, carbapenems and vancomycin is prohibitive. People in developing countries usually cannot even consider these drugs for economic reasons. For example, drug-resistant TB is many times costlier to treat than susceptible TB (Cohen 1992). Clostridium difficile and Methicillin-resistant staphylococcus aureus (MRSA) infections kill ten thousands every year in the US alone (CDC 2013). No wonder that healthcare is forming bigger chunks of government budgets by the year.
The direct public health ramifications of antibiotic resistance are truly enormous with estimated losses in hundreds of millions of lives and trillions of dollars when accounting for prolonged illness, death, production losses and negative impacts on food security and livelihoods. Every year 700,000 people might be dying from drug resistant infections worldwide and by 2050, this figure would rise to 10 million. Global Gross Domestic Product (GDP) could be reduced by 2 to 3.5% (O’Neill 2016). Calculations by World Bank experts pegged annual adverse global GDP impact at 1.0 to 3.2% by 2030 ($1 to 3.4 trillion) or 1.1% to 3.8% by 2050 ($2 to 6.1 trillion) as a consequence of shocks to the labor supply. Measured in 2007 US dollars and discounted at a reasonable 3.5% annually, even the ‘optimistic’ figure estimate is unsettling (World Bank 2017).
Causes: Animal Agriculture and Aquaculture Use
These dire figures illustrate why governments in Europe banned the use of antibiotics for growth promotion in livestock and also imposed other restrictions on use. This highly controversial practice involves dosing animals with low concentration antibiotics, also called sub therapeutic doses, and enables animals to achieve faster growth on less feed (Witte 1998) even though the reasons for this are not well understood (Page and Gautier 2012). Yet, the benefits are more marked in farms with poorer hygiene practices indicating that antibiotics could be supplanted or substituted by more effective animal management (J. Rushton 2015). Even the economic benefits are disputable after considering the costs of antibiotics (Graham, Boland, and Silbergeld 2007). Therapeutic use of antibiotics in animals involves many of the same classes of drugs as used in human treatment. However, therapeutic use also includes mass administration of antibiotics to healthy animals, often in their feed, as a preventive measure (prophylactic use) or mass administration of antibiotics to an entire group of animals when only some of them are infected (metaphylactic use) (Urban-Chmiel and Grooms 2012) thereby fostering resistance. The widespread indiscriminate therapeutic use of antibiotics in aquaculture is also alarming because aquatic environments are especially good for the proliferation of resistant bacteria (FAO 2005; Salyers 1995; Marshall and Levy 2011).
Exact data on antibiotic usage in agriculture is not available for most countries but we can estimate that the numbers are enormous in both developed and developing nations. In USA, between 30 to 80% of all antibiotics, are used on animals (Vogt and Jackson 2001; Kahn 2016; Jonathan Rushton, Ferreira, and Stärk 2014). The livestock industry has been lobbying for decades to prevent restrictions on antibiotic usage citing both the lack of irrefutable evidence and economic concerns. The European experience lends them some weight. European pork producers probably lost €870 million annually due to the ban on growth promoting antibiotics because the competing US pork industry faced no ban and consequently did not incur the additional administration costs incurred. The ban also resulted in many smaller farms going out of business. The effects of the ban on the development of resistance in humans were altogether insignificant even though it did discernibly lead to lowered VRE resistance in animals and lower transmission of some resistant zoonotic (animal to human) diseases (Kahn 2016). The industry has actively blockaded research on actual animal farms in the US (Moyer 2016). It has also talked much about the relative absence of antibiotic residues in animal food while completely ignoring the bigger problem of residues in manure and sewage releasing drug resistant strains in the environment.
There is incontrovertible evidence that resistant bacteria get transferred to livestock handlers and regularly cause zoonotic diseases (Marshall and Levy 2011). The resistance causing effect of sub therapeutic doses has been known for long (ter Kuile, Kraupner, and Brul 2016; Levy, Fitzgerald, and Macone 1976). There is also strong genetic evidence for the livestock origin of certain forms of resistance such as the tracing the livestock use of avoparcin to vancomycin resistant enterococci (VRE) (Witte 2000) as well as strong correlations to resistance outbreaks in humans (Marshall and Levy 2011). Resistance origin does not even require the identical drug to be used in animals (Jonathan Rushton, Ferreira, and Stärk 2014). Nonetheless, while resistant strains of zoonotic bacteria such as Campylobacter, Salmonella or E. Coli are found annually in inspections of retail meats, proving the farm to fork connection has mostly proved elusive due to a dearth of studies that cover the entire supply chain (McCrackin et al. 2016; Marshall and Levy 2011).
Solutions
There are limitations to the introduction of new antibiotics which mean that we ought to preserve the use of existing classes (Cormican and Vellinga 2012). Rapid diagnostics would be of great help by helping a doctor prescribe the right antibiotic. Besides, research on other promising forms of treatment including bacteriophages (viruses targeting specific bacteria), improving human immunity and intravenous immunoglobulin treatment need to be pursued (Marston et al. 2016). The effect on non-antibiotic substances such as heavy metals on resistance too needs further study (Salyers 1995) as does the spread of diseases from companion animals such as dogs (J. Rushton 2015; Kahn 2016). Drug companies also lack the economic incentives to pursue the development of new antibiotics due to a combination of higher costs of drug development, tougher approval standards, failures, and uncertain period of effective use (Marston et al. 2016; Hoelzer et al. 2017). Vaccines are economically even less attractive. Very few new antibiotics and vaccines are currently in the pipeline. Clearly, continual subsidies or incentives are needed to promote antibiotic and vaccine development.
Antibiotics in animal agriculture and aquaculture need to be severely restricted based on the precautionary principle wherein action is taken in the interests of larger public health even if a relation is unproven at present. Bacterial gene transfer can clearly occur in human intestinal systems after consumption of food with resistant bacteria (Salyers 1995). Furthermore, the impact on food security is likely to be little as the growth benefits are in the low single digits (Witte 1998), and less stressful housing could reduce therapeutic need as well. Economic incentives for farmers could counter higher management costs. Surely, savings from healthcare costs due to lower levels of resistant bacteria in meat alone may amount to billions (Kahn 2016). At a time when the massive environmental impacts of the livestock industry are well understood (Steinfeld et al. 2006), it would be more prudent to try to save part of the animal products wasted. For example, 22% meat, 50% seafood and 20% milk are wasted in America (Gunders 2012). Gambling on the future of billions because of inadequate quantification of risks versus benefits of surplus meat is utterly irresponsible, to say the least.
Looking at the Biggest Cause and Solution
However, the biggest preventable factor relates to clinical misuse of antibiotics and poor hygiene practices. Half of the antibiotics commonly prescribed are unnecessary or outrightly incorrect (Kahn 2016). Knowingly prescribing antibiotics for viral infections is indeed unconscionable even if patients expressly demand them. Perhaps some legal protection is needed for doctors fearing litigation. The widespread sales of spurious drugs or those with lower active ingredients in developing countries needs to be nipped in the bud (Yadav and Rawal 2015; WHO 1999b, 1999a; Kelesidis and Falagas 2015). Poorly informed physicians often prescribe second or third level antibiotics when first level would suffice. In India, people frequently counter infections caused by poor sanitary habits such as open defecation with antibiotics, a practice so egregious that the New York Times singled it out as a cover story (Harris 2014). It is little wonder that the pan-resistant NDM strain, which is now spreading worldwide, evolved in India (The Guardian 2019; Kelland and Hirschler 2010). Even in USA, hand-hygiene compliance in hospitals is often less than 50% (Kirkland et al. 2012). This really needs to change.
Already, hospital acquired infections (HAI) are undermining the confidence of patients in hospitals because they have become notorious for disease transfer. Procedures causing suppressed immunity such as transplants, chemotherapy, bypass surgeries etc. have become more common and such patients are the most vulnerable; they are most likely to harbour and subsequently transmit resistant bacteria. Pathogens adhere persistently to surfaces such as plastic catheters and drug resistant HAIs often kill healthy patients who came to a hospital for a routine procedure. It will be a great public health loss if people start avoiding these procedures although avoiding often unnecessary surgeries such as Caesarians might turn out to be beneficial.
Today, antibiotic resistance is recognized as one of the top health challenges of the 21st Century by leading world health organizations. The World Health Organization initiated a Global Action Plan in 2015 with the intention to create country specific and time bound targets (WHO 2015). A ‘One Health Approach’ that seeks to treat antibiotics as a global common good and considers human health, animal health and the environment together is likely to yield the best results. International standardization of surveillance data, methodologies and regulations is sorely needed. Resistant bugs will continue to travel with people and goods in a globalized world and so protective measures merely implemented in some developed countries would not suffice. Measures such as antimicrobial stewardship programs that seek to optimize drug usage while inducing minimal resistance are now being adopted in nations like the UK (Robb and Seaton 2016). This is timely as strong evidence suggests that once resistance originates, resistance persists in the population even after the pressure of antibiotic use is removed (Levin et al. 1997). Delaying the initial onset of resistance is crucial.
Encouragingly, China has already adopted the world’s toughest regulatory measures to combat this scourge (Kahn 2016). Other developing nations would do well to follow. They would also do well to invest in mitigation efforts. An investment of just $9 billion annually improving core veterinary and public health systems in developing countries may yield between 10 to 27 trillion dollars in net present value if just 10% of mitigation efforts are successful (expected economic rate of return is between 31 to 88%). If developed countries invest this capital, their returns are even higher (World Bank 2017). In the world of finance, it does not get much better than that.
Citations
Bhullar, Kirandeep, Nicholas Waglechner, Andrew Pawlowski, Kalinka Koteva, Eric D. Banks, Michael D. Johnston, Hazel A. Barton, and Gerard D. Wright. 2012. “Antibiotic Resistance Is Prevalent in an Isolated Cave Microbiome.” Edited by Ramy K. Aziz. PLoS ONE 7 (4): e34953.
https://doi.org/10.1371/journal.pone.0034953.
CDC. 2013. “Antibiotic Resistance Threats in the United States.” CDC Publications, 1–114.
Cohen, Mitchell.L. 1992. “Epidemiology of Drug Resistance: Implications for a Post-Antimicrobial Era.” Science. 257 (5073): 1050–5.
https://www.sciencemag.org/site/feature/data/diseases/PDFs/257-5073-1050.pdf.
Cormican, Martin, and Akke Vellinga. 2012. “Existing Classes of Antibiotics Are Probably the Best We Will Ever Have.” BMJ (Online) 344 (7857): e3369.
https://doi.org/10.1136/bmj.e3369.
D’Costa, Vanessa M., Christine E. King, Lindsay Kalan, Mariya Morar, Wilson W. L. Sung, Carsten Schwarz, Duane Froese, et al. 2011. “Antibiotic Resistance Is Ancient.” Nature 477 (7365): 457–61.
https://doi.org/10.1038/nature10388.
Falagas, Matthew E, Ioannis A Bliziotis, Sofia K Kasiakou, George Samonis, Panayiota Athanassopoulou, and Argyris Michalopoulos. 2005. “Outcome of Infections Due to Pandrug-Resistant (PDR) Gram-Negative Bacteria.” BMC Infectious Diseases 5 (1): 24.
https://doi.org/10.1186/1471-2334-5-24.
FAO. 2005. “Responsible Use of Antibiotics in Aquaculture.” Rome.
http://www.fao.org/3/a-a0282e.pdf.
Graham, Jay P, John J Boland, and Ellen Silbergeld. 2007. “Growth Promoting Antibiotics in Food Animal Production: An Economic Analysis.” Public Health Reports 122 (1): 79–87.
https://doi.org/10.1177/003335490712200111.
Gunders, Dana. 2012. “Wasted: How America Is Losing up to 40 Percent of Its Food from Farm to Fork to Landfill.” NRDC Issue Paper.
Harris, Gardiner. 2014. “Poor Sanitation in India May Afflict Well-Fed Children With Malnutrition.” The New York Times, 2014.
Hoelzer, Karin, Nora Wong, Joe Thomas, Kathy Talkington, Elizabeth Jungman, and Allan Coukell. 2017. “Antimicrobial Drug Use in Food-Producing Animals and Associated Human Health Risks: What, and How Strong, Is the Evidence?” BMC Veterinary Research 13 (1): 211.
https://doi.org/10.1186/s12917-017-1131-3.
Kahn, Laura H. 2016. One Health and the Politics of Antimicrobial Resistance. Baltimore: John Hopkins University Press.
Kelesidis, Theodoros, and Matthew E Falagas. 2015. “Substandard/Counterfeit Antimicrobial Drugs.” Clinical Microbiology Reviews 28 (2): 443–64.
https://doi.org/10.1128/CMR.00072-14.
Kelland, Kate, and Ben Hirschler. 2010. “Scientists Find New Superbug Spreading from India | Reuters.” Reuters.Com. 2010.
Kirkland, Kathryn B, Karen A Homa, Rosalind A Lasky, Judy A Ptak, Eileen A Taylor, and Mark E Splaine. 2012. “Impact of a Hospital-Wide Hand Hygiene Initiative on Healthcare-Associated Infections: Results of an Interrupted Time Series.” BMJ Quality & Safety 21 (12): 1019–26.
https://doi.org/10.1136/bmjqs-2012-000800.
Kuile, Benno H. ter, Nadine Kraupner, and Stanley Brul. 2016. “The Risk of Low Concentrations of Antibiotics in Agriculture for Resistance in Human Health Care.” Edited by Hermann Heipieper. FEMS Microbiology Letters 363 (19): fnw210.
https://doi.org/10.1093/femsle/fnw210.
Levin, B R, M Lipsitch, V Perrot, S Schrag, R Antia, L Simonsen, N M Walker, and F M Stewart. 1997. “The Population Genetics of Antibiotic Resistance.” Clinical Infectious Diseases : An Official Publication of the Infectious Diseases Society of America 24 Suppl 1 (January): S9-16.
http://www.ncbi.nlm.nih.gov/pubmed/8994776.
Levy, Stuart B., George B. Fitzgerald, and Ann B. Macone. 1976. “Spread of Antibiotic-Resistant Plasmids from Chicken to Chicken and from Chicken to Man.” Nature 260 (5546): 40–42.
https://doi.org/10.1038/260040a0.
Magiorakos, A.-P., A. Srinivasan, R.B. Carey, Y. Carmeli, M.E. Falagas, C.G. Giske, S. Harbarth, et al. 2012. “Multidrug-Resistant, Extensively Drug-Resistant and Pandrug-Resistant Bacteria: An International Expert Proposal for Interim Standard Definitions for Acquired Resistance.” Clinical Microbiology and Infection 18 (3): 268–81.
https://doi.org/10.1111/j.1469-0691.2011.03570.x.
Marshall, Bonnie M., and Stuart B. Levy. 2011. “Food Animals and Antimicrobials: Impacts on Human Health.” Clinical Microbiology Reviews 24 (4): 718–33.
https://doi.org/10.1128/CMR.00002-11.
Marston, Hilary D., Dennis M. Dixon, Jane M. Knisely, Tara N. Palmore, and Anthony S. Fauci. 2016. “Antimicrobial Resistance.” JAMA 316 (11): 1193.
https://doi.org/10.1001/jama.2016.11764.
McCrackin, M.A., Kristi L. Helke, Ashley M. Galloway, Ann Z. Poole, Cassandra D. Salgado, and Bernadette p. Marriott. 2016. “Effect of Antimicrobial Use in Agricultural Animals on Drug-Resistant Foodborne Campylobacteriosis in Humans: A Systematic Literature Review.” Critical Reviews in Food Science and Nutrition 56 (13): 2115–32.
https://doi.org/10.1080/10408398.2015.1119798
Michael, Shnayerson, and Mark J. Plotkin. 2002. The Killers Within: The Deadly Rise Of Drug-Resistant Bacteria. 1st ed. Boston; London: Little, Brown and Co.
https://newcatalog.library.cornell.edu/catalog/4398249.
Moyer, Melinda Wenner. 2016. “The Looming Threat of Factory Superbugs.” Scientific American 315 (6): 70–79.
https://doi.org/10.1038/scientificamerican1216-70.
NY Times. 1945. “PENICILLIN’S FINDER ASSAYS ITS FUTURE; Sir Alexander Fleming Says Improved Dosage Method Is Needed to Extend Use Other Scientists Praised Self-Medication Decried.” New York Times, June 26, 1945.
O’Neill, J. 2016. “Antimicrobial Resistance : Tackling a Crisis for the Health and Wealth of Nations.” Review on Antimicrobial Resistance, no. December: 1–16.
https://doi.org/10.1038/510015a.
Page, S.W., and P. Gautier. 2012. “Use of Antimicrobial Agents in Livestock.” Revue Scientifique et Technique de l’OIE 31 (1): 145–88.
https://doi.org/10.20506/rst.31.1.2106.
Robb, Fiona, and Andrew Seaton. 2016. “Components of an Antimicrobial Stewardship Programme.” In Antimicrobial Stewardship, edited by Matthew Laundy, Mark Gilchrist, and Laura Whitney, First, 12–19. New York: Oxford University Press.
https://global.oup.com/academic/product/antimicrobial-stewardship-9780198758792?cc=us&lang=en&.
Rushton, J. 2015. “Anti-Microbial Use in Animals: How to Assess the Trade-Offs.” Zoonoses and Public Health 62 (April): 10–21.
https://doi.org/10.1111/zph.12193.
Rushton, Jonathan, Jorge Pinto Ferreira, and Katherine D.C. Stärk. 2014. “The Use of Antimicrobials in the Livestock Sector.” OECD Food, Agriculture and Fisheries Papers No. 68. Paris.
https://doi.org/10.1787/5jxvl3dwk3f0-en.
Salyers, Abigail A. 1995. Antibiotic Resistance Transfer in the Mammalian Intestinal Tract. Austin: R.G. Landes Co.
Steinfeld, H, P Gerber, T Wassenaar, V Castel, M Rosales, and C de Haan. 2006. “Livestock’s Long Shadow: Environmental Issues and Options.” Renewable Resources Journal 24 (4): 15–17.
The Guardian. 2019. “Genes Linked to Antibiotic-Resistant ‘India Superbug’ Found in Arctic | South China Morning Post.” South China Morning Post. 2019.
Urban-Chmiel, R., and D L Grooms. 2012. “Prevention and Control of Bovine Respiratory Disease.” J. Livestock Sci 3: 27–36.
https://livestockscience.in/wp-content/uploads/2012/Bovine_Respiratory_Disease.pdf.
Vogt, Donna U., and Brian A. Jackson. 2001. Antimicrobial Resistance: An Emerging Public Health Issue. Edited by Susan Boriotti and Donna Dennis. New York: Novika Books.
WHO. 1999a. “Counterfeit and Substandard Drugs in Myanmar and Viet Nam. Report of a Study Carried out in Cooperation with the Governments of Myanmar and Viet Nam.” World Health Organization.
http://apps.who.int/medicinedocs/pdf/s2276e/s2276e.pdf.
WHO. 1999b. “Summary of WHO Counterfeit Drug Database as of April 1999, Unpublished Paper of the WHO Division of Drug Management and Policies.” Geneva.
WHO. 2015. Global Action Plan on Antimicrobial Resistance. Geneva: World Health Organization. www.paprika-annecy.com.
Witte, Wolfgang. 1998. “Medical Consequences of Antibiotic Use in Agriculture.” Science 279 (5353): 996–98.
http://go.galegroup.com/ps/i.do?p=AONE&u=nysl_sc_cornl&id=GALE%7CA20397612&v=2.1&it=r&sid=summon.
WHO. 2000. “Selective Pressure by Antibiotic Use in Livestock.” International Journal of Antimicrobial Agents 16 (November): 19–24.
https://doi.org/10.1016/S0924-8579(00)00301-0.
World Bank. 2017. “Drug-Resistant Infections : A Threat to Our Economic Future : Final Report (English).” Washington, D.C.
http://documents.worldbank.org/curated/en/323311493396993758/final-report.
Yadav, Sankalp, and Gautam Rawal. 2015. “Counterfeit Drugs: Problem of Developing and Developed Countries.” International Journal of Pharmaceutical Chemistry and Analysis 2 (1): 46–50.
Abhinav Choudhry
Abhinav Choudhry is a currently working with Tata Cornell Institute at the Cornell SC Johnson College of Business, Cornell University on topics at the intersection of agriculture and its relationship to public health. Abhinav graduated with a Master of Public Administration degree from Cornell University with a concentration in Environmental Policy and also completed a fellowship program in Environmental Finance and Impact Investing. In 2017, he worked with the Environmental Defense Fund in California, the USA on projects aimed at finding conservation finance solutions for mitigating greenhouse gas pollution and agricultural runoff. He has also consulted for a startup solar energy company in Nigeria in 2018.
Abhinav has a Masters in Finance & Control from the University of Delhi and a Bachelors in Computer Science Engineering. Before concentrating on issues relating to sustainable development, he accrued over four years of experience in finance in the private sector, working with Citibank and Nestle in India.
Recent Articles
- Creating a future-proof curriculum for the digital age
- Policymakers, wake up! Floods in Chennai are not Alarm bells, they are explosions
- 'Environmental Finance — Private Capital and Private Profits for Public Gain— Pretty Promising, though not without Pain'
- Inclusive Innovations in Education Praxis
- Antibiotic Resistance: Fighting a Multi-Headed Demon